
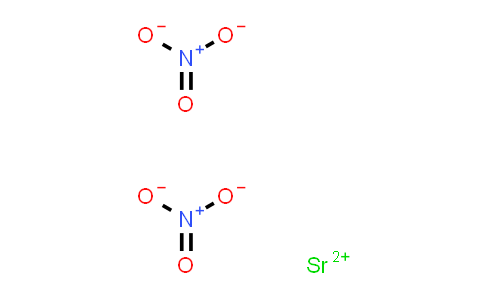
The accurate measurement of iron ion concentrations in a glass is a strong indicator of the oxidation state of the glass and hence the quality of the glass. In embodiments of the present invention, oxygen levels in a glass material are reflected by the relative amounts of Fe(II) and Fe(III) that exist in the glass material and in particular, by the ratio of Fe(III) to Fe(II) in the glass material. Against this backdrop the present invention has been developed. There is also a need in the industry for fast, efficient, and accurate methods to avoid producing and shipping unacceptable glass product, and where appropriate, a need to make this determination on site where the glass is manufactured, thereby minimizing the time it takes to make a corrective action. This is especially true for glass articles going to the highly demanding FPDs market. As a result, there is a need in the industry to be able to accurately follow the iron ion levels, and thereby the oxidation states in the manufactured glass material. Therefore, either feedback time is slowed and/or quality of the feedback information is unreliable to the glass technologist or process engineer, making it difficult to make corrective actions within the glass melting process. Unfortunately, existing techniques for determining the iron oxidation states in the glass either require expensive and time consuming chemical analysis, for example, Atomic Absorption Spectroscopy (AAS) or Electron Paramagnetic Resonance (EPR) or require less accurate spectrophotographic methods (focused on two wavelengths to monitor a glass property, e.g., 300-400 nm and 1000-1200 nm), which although much quicker are often inconsistent. The oxidation state of the glass has previously been determined to be reflected in the relative amounts of the iron ions Fe(II) and Fe(III) that exist as tramp components in most manufactured glass.

The addition of an external light source then completes the display. This can either be done intrinsically (passive matrix Liquid Crystal Display) or actively through the creation of a thin film transistor (TFT) at each pixel location (Active Matrix Liquid Crystal Display, AMLCD). Pixelation necessary for a true display is done by applying the necessary voltage to only a small area of the total liquid crystal. By applying polarizing films to the outside of this glass-liquid crystal glass “cell”, a voltage-controlled valve may be created. Liquid crystal materials encompass a range of organic materials that have the properties of being birefringent (refractive index dependent upon the crystal orientation) and having an ability to switch crystal orientations by the application of an electric field. In LCDs, two sheets of glass, each on the order of 1 mm thick, surround a thin, 3-5 &mgr m, layer of liquid crystal material. One well-known member of this family of displays is the Liquid Crystal Display (LCD). A relatively new, and demanding, use of glass is as a substrate for Flat Panel Displays (FPDs).
